Distributed Wind
Resource Categorization
The U.S. Department of Energy's (DOE's) Wind Energy Technologies Office defines distributed wind in terms of technology application, based on a wind plant's location relative to end-use and power distribution infrastructure, rather than technology or project size. The following wind system attributes are used by the office to characterize them as distributed:
- Proximity to End-Use: wind turbines that are installed at or near the point of end use to meet on-site energy demand or support the operation of the existing distribution grid
- Point of Interconnection: wind turbines that are connected on the customer side of the meter (also known as behind-the-meter), are connected directly to the distribution grid, or are off-grid in a remote location.
Distributed wind energy systems are commonly installed on, but are not limited to, residential, agricultural, commercial, industrial, and community sites, and can range in size from a 1-kw turbine at a home to multimegawatt turbines at a manufacturing facility or connected to a local distribution system.
Distributed wind project performance and cost is represented using four turbine technology classes: residential, commercial, midsize, and large. When used in the context of wind turbine technology, these labels refer specifically to the size of the turbines. Notably, any turbine size may be installed at the consumer’s site, independent of sector (residential, commercial, or industrial), with the applicability of a specific turbine being governed by both the ability to site a given machine on a specific parcel of land and the consumer’s load (Lantz et al., 2016).
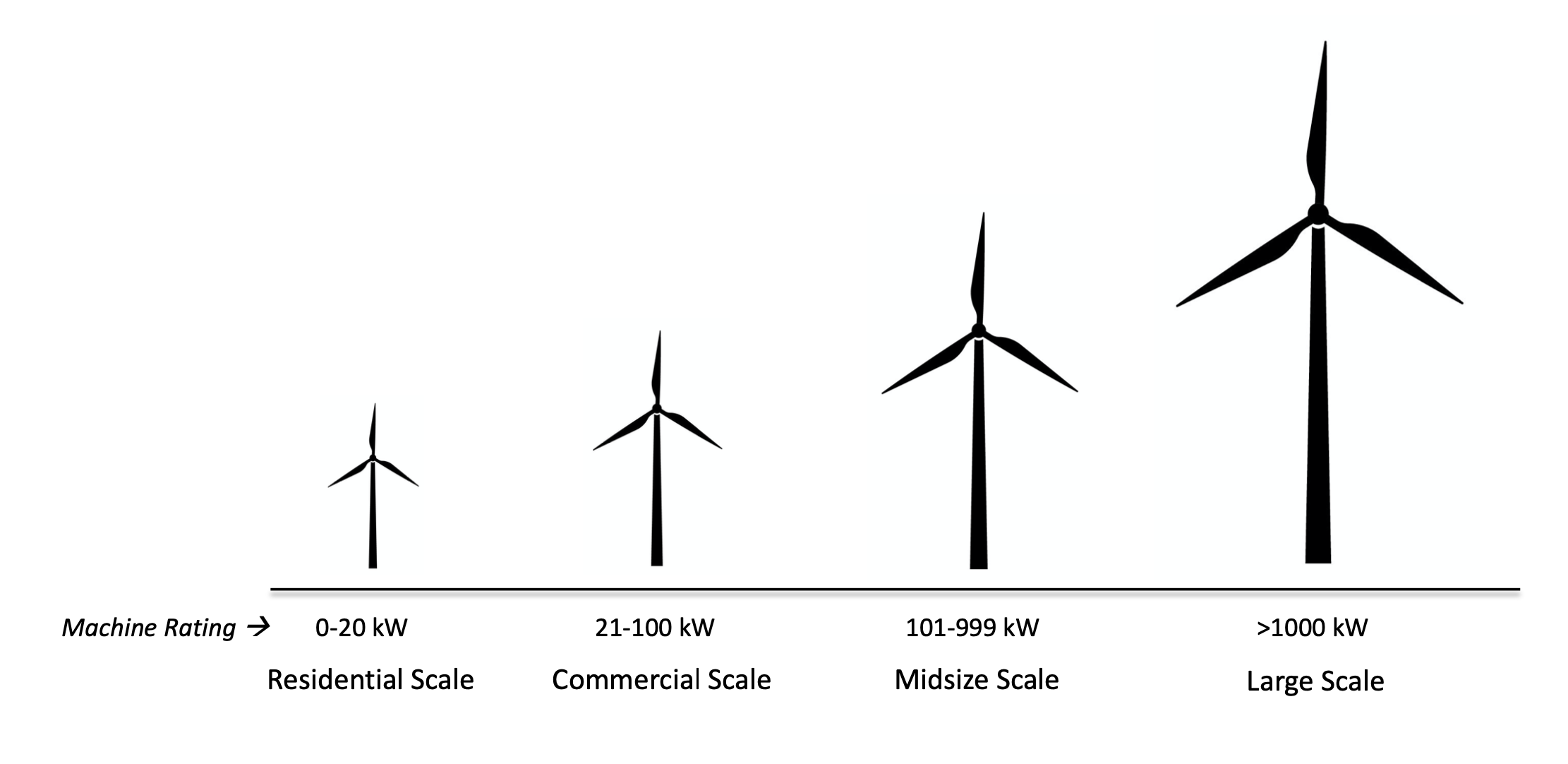
Turbine Technology Class | Size Range (kW) | Hub Height (m) |
Residential | < 20 | 35 |
Commercial | 21–100 | 45 |
Midsize | 101–999 | 55 |
Large | >1,000 | 80 |
For distributed wind, similar to land-based utility-scale wind, each of the potential wind sites characterized in the ATB is associated with one of 10 wind speed classes. The following table shows each resource class as well as the resulting mean wind speed ranges that define each class. Wind classes 1-10 sweep an exhaustive range of average annual wind speeds that distributed wind projects can be expected to have in the contiguous US. Wind Speed Class 1 is suggestive of a resource-rich wind resource that is most attractive for wind project development, and Wind Speed Class 10 represents a less favorable wind resource site.
Wind Class | Average Annual Wind Speed @ 110m Above Ground | Sheer | Weibull K Factor |
---|---|---|---|
1 | 9.52 | 0.14 | 2 |
2 | 8.87 | 0.14 | 2 |
3 | 8.66 | 0.14 | 2 |
4 | 8.45 | 0.14 | 2 |
5 | 8.2 | 0.14 | 2 |
6 | 7.84 | 0.14 | 2 |
7 | 7.36 | 0.14 | 2 |
8 | 6.8 | 0.14 | 2 |
9 | 6.21 | 0.14 | 2 |
10 | 5.13 | 0.14 | 2 |
Scenario Descriptions
Given the highly localized nature of the technoeconomic performance of a distributed wind project, estimating future capital costs and other trends is challenging. The current status of the industry coupled with substantial uncertainty in terms of future demand and deployment at the global level add to the challenge. The costs modeled here reflect an initial assessment, but also a rigorous analytical estimate. Given the substantial uncertainty in this domain, a broad range of values is created to support scenario analysis.
For large turbines, we rely on the Low Wind Cost LCOE trajectory detailed by Wind Vision 2015 and in the NREL's ATB. This independent LCOE trajectory is used for large wind turbines, which have seen significant public and private sector research and development investment over the past three decades and are believed to have captured a more substantial portion of their long-term cost reduction potential in today’s modern technology. This trajectory results in substantially less change in 2050 LCOE than the various trajectories applied to the other distributed wind size classes. Notably, although we use the LCOE trajectory from Wind Vision 2015 for the large turbine sizes, we do not attempt to use the specific LCOE input values (e.g., capital cost, O&M, and capacity factors) detailed in DOE’s Wind Vision (2015). Rather, we use the characterizations noted earlier and calculated the requisite capital cost required to achieve the specified LCOE reduction. DOE’s Low Wind Cost trajectory (DOE and NREL, 2015) is applied for two reasons: it is more consistent with subsequent analysis and research on future wind turbine cost trajectories (Wiser and Bolinger, 2016), and it is assumed that distributed wind applications have opportunities for innovation and efficiency in development and balance-of-station costs that may increase their probability of relatively greater cost reduction than modern multimegawatt wind power plants (Bhaskar and Stehly, 2021). A summary of the CAPEX reductions between 2020 and 2030 is provided in the table below (Summary of Technology Innovation by Scenario).
Scenario | Description | Rationale |
---|---|---|
Conservative |
| The reductions are derived from land-based wind median learning rate, projected global growth rates, short-term cost estimates, and the Wind Vision study cost reduction assumptions (DOE and NREL, 2015) |
Moderate |
| |
Advanced |
|
Scenario | Rotor, Nacelle Assembly | Tower | Others |
---|---|---|---|
Conservative | Same as land-based wind. | Same as land-based wind. | |
Moderate | Same as land-based wind. | Same as land-based wind. | specific power reduction standardized zoning, permitting, interconnection, and incentives higher volume of turbine manufacturing leading to lower overhead charged per turbine |
Advanced | Same as land-based wind. | Same as land-based wind. | specific power reduction standardized zoning, permitting, interconnection, and incentives higher volume of turbine manufacturing leading to lower overhead charged per turbine |
The reductions are derived from land-based wind median learning rate, projected global growth rates, short-term cost estimates, and the Wind Vision study cost reduction assumptions (DOE and NREL, 2015) |
Representative Technology
Analysis results for distributed wind detailed in the ATB are contingent on a detailed characterization or representation of distributed wind technologies. Even estimates of addressable resource potential require knowledge of potential hub heights and energy generation for wind turbines ranging from kilowatt- to megawatt-scale. The technology characterizations, such as turbine rating, hub height, and rotor diameter for the baseline scenarios, are summarized in the following table. These characteristics represent recent (2019) technology, as informed by empirical data and certified wind turbine equipment (Bhaskar and Stehly, 2021).
To obtain current and future cost and performance estimates, the technology representations for the Base Year (2020) and 2030 (future) are defined. For a detailed breakdown of the current and future distributed wind turbine performances including their representative power curves (current and future), see Appendix B of the DWFS 2022 (McCabe et al., 2022). The turbine performance improvements up till year 2050 remain the same across all 3 scenarios (Conservative, Moderate, Advanced). Existing literature on the future performance improvements of distributed wind turbines do not breakdown the performance improvements by various scenarios, resulting in single performance improvement trajectories for all 4 turbine classes (DOE and NREL, 2015) and (Lantz et al., 2016). In contrast to land-based utility-scale wind, distributed wind projects tend to be single turbine projects (though projects can have more than 1 turbine). The technological advances seen in multi-turbine utility scale projects primarily revolve around advances in plant-level improvements in energy production owing to plant level design and optimization. As a result, most technological innovations in utility-scale wind do not translate to distributed wind projects.
Project Scale Classification | Turbine Rating (kW) | Hub Height (m) | Rotor Diameter (m) | Specific Power | Max Coefficient of Performance (Cp) | Max Tip Speed (m/s) | Max Tip-Speed Ratio |
---|---|---|---|---|---|---|---|
Residential | 20 | 35 | 10 | 166 | 0.4 | 95 | 9.7 |
Commercial | 100 | 45 | 24 | 167 | 0.5 | 75 | 8 |
Midsize | 650 | 55 | 47 | 169 | 0.5 | 70 | 8 |
Large | 1,500 | 80 | 77 | 167 | 0.5 | 82 | 8 |
Project Scale Classification | Turbine Rating (kW) | Hub Height (m) | Rotor Diameter (m) | Specific Power | Max Coefficient of Performance (Cp) | Max Tip Speed (m/s) | Max Tip-Speed Ratio |
---|---|---|---|---|---|---|---|
Residential | 20 | 35 | 10 | 150 | 0.4 | 95 | 9.7 |
Commercial | 100 | 45 | 24 | 150 | 0.5 | 95 | 9.7 |
Midsize | 650 | 55 | 47 | 150 | 0.5 | 95 | 9.7 |
Large | 1,500 | 80 | 77 | 150 | 0.5 | 95 | 9.7 |
Methodology
This section describes the methodology to estimate current and future CAPEX, O&M, and capacity factor. The current and future cost and performance estimates assume a single turbine project across all 4 turbine technology classes, which is consistent with current project sizes seen in literature (Bhaskar and Stehly, 2021). To resolve the substantial uncertainty in future capital cost estimates for distributed wind applications, we develop an analytical method that forms the basis for our estimates. For residential, commercial, and midsize turbine classes, we use a combination of technology learning rates and estimated global growth rates to identify potential changes in estimated LCOE (Lantz et al., 2016). The resulting LCOE trajectories are used in conjunction with future performance and O&M characterizations and constant financing terms to calculate capital costs (McCabe et al., 2022).
Capital Expenditures (CAPEX)
For the future CAPEX projections of distributed wind projects, we use the same approach to modeling future cost projections of distributed wind under the various scenarios discussed in (Lantz et al., 2016), but for updated year 2020 costs obtained from NREL’s 2020 Cost of Wind Energy study (Stehly and Duffy, 2022) and the Distributed Wind Futures Study (McCabe et al., 2022).
Components of the CAPEX for a representative distributed wind project of any scale are summarized in (McCabe et al., 2022) and (Bhaskar and Stehly, 2021).
Cost Type | Cost Component |
---|---|
Turbine CAPEX | Turbine (price to installer) |
Tower (price to installer) | |
Other turbine equipment costs | |
Original equipment manufacturer's extended warranty | |
Transport to customer | |
Balance-of-system CAPEX | Zoning, permitting, interconnection, and incentives |
Engineering and design | |
Management (e.g., project and construction management) | |
Turbine foundation | |
Site preparation | |
Grid connection | |
Collection system | |
Turbine erection and installation | |
Development costs |
In the ATB, CAPEX reflects typical plants and does not include differences in regional costs associated with labor, materials, taxes, or system requirements. The CAPEX estimations calculated here are for single-turbine projects across all four project scales. Distributed wind projects range from 1 kW in size to multimegawatt turbines. Accordingly, distributed wind projects can have multiple turbines within the same project, although the likelihood of multiple turbines in a project is higher for midsize and large-scale projects (as opposed to residential and commercial-scale projects). Estimating the cost of multi-turbine distributed wind projects is beyond the scope of this ATB analysis. For a detailed assessment of multi-turbine midsize and large projects, refer to (Bhaskar and Stehly, 2021).
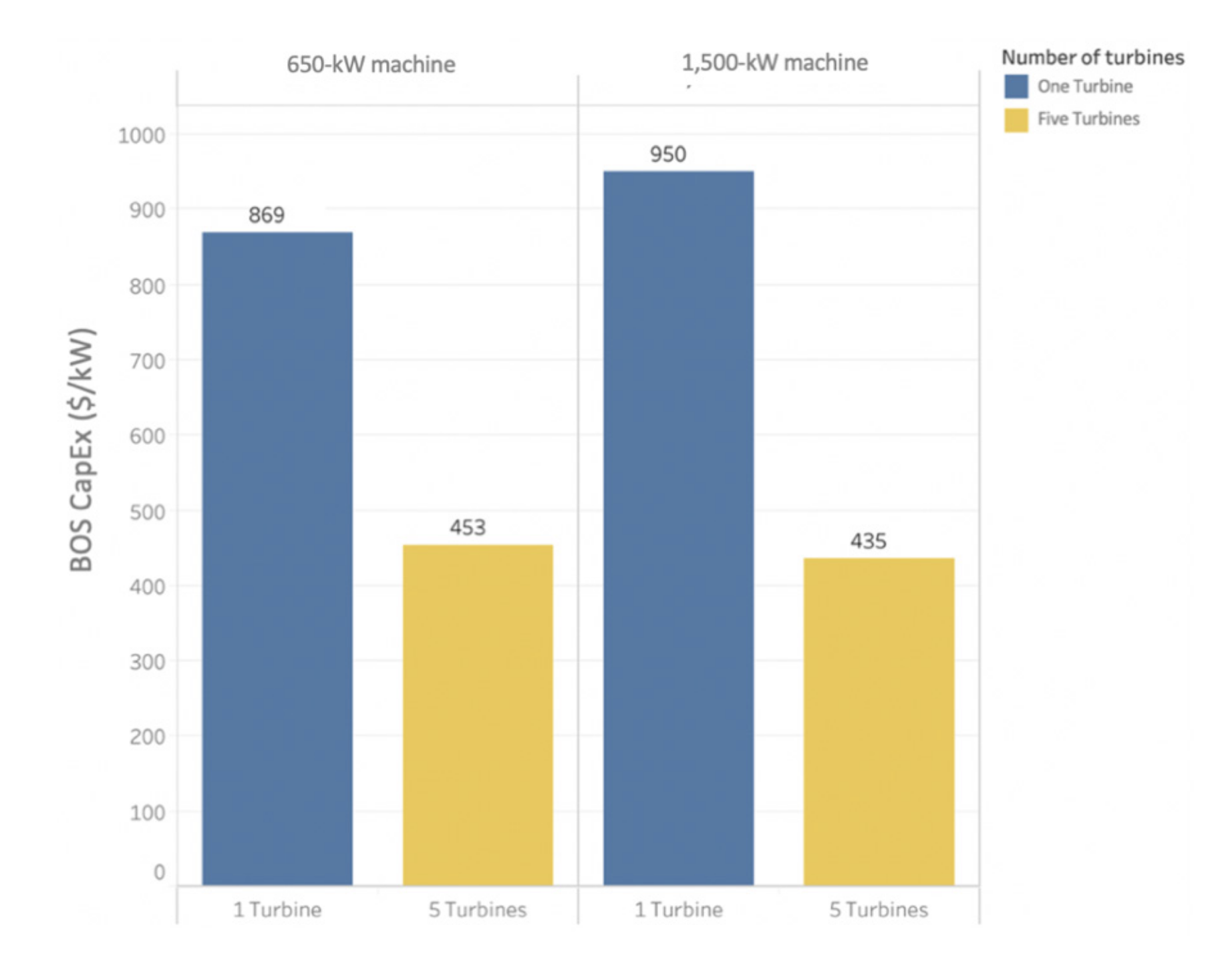
Source: (Bhaskar and Stehly, 2021)
Operation and Maintenance (O&M) Costs
Definition: Operation and maintenance (O&M) costs represent the all-in fixed and variable expenditures required to operate and maintain a distributed wind project. For a detailed breakdown of the components of the O&M cost bucket, review the O&M section of the land-based wind page of the ATB.
Base Year: The all-in O&M of $35/kW-yr in the Base Year for all four project scales is reported in the 2020 Cost of Wind Energy Review (Stehly and Duffy, 2022).
Future Years: O&M costs are assumed to decline consistently across turbine sizes by a cumulative 10% (an annual rate of approximately 0.3% per year) between 2014 and 2050, consistent with the Wind Vision (DOE and NREL, 2015), for all scenarios that assume a change in distributed wind O&M.
Capacity Factor
Definition: Similar to land-based wind, the capacity factor for a distributed wind project is influenced by the project's generation profile, expected downtime, and energy losses within the wind plant. The specific power (i.e., ratio of machine rating to rotor swept area), hub height, and use of stall regulated or pitch regulated machine are design choices that influence the capacity factor.
To calculate the Base Year and future capacity factors, the 2020 and 2030 (and beyond), turbine characteristics are defined in the Representative Technology section are input into the System Advisor Model (SAM) to develop an idealized power curve, and SAM is run for each of the weighted average wind speeds in each wind speed class. The capacity factors are calculated at the representative turbine hub height by extrapolating the wind speed up or down from the referenced 110-m, above-ground-level, long-term average hourly wind resource data from the Wind Integration National Dataset (WIND) Toolkit.
The following chart shows a range of capacity factors based on variation in the resource for distributed wind projects in the contiguous United States and the future capacity factor estimates for the Conservative, Moderate, and Advanced scenarios.
Future Trends
Future performance of distributed wind technologies is characterized using two primary assumptions. First, we assume turbine-specific power will continue to decline to levels of approximately 150 watts per square meter (W/m2). Although future specific power estimates will ultimately be determined by the relative cost of additional blade length and rotor growth to additional generator capacity along with the anticipated time spent operating at maximum power, the 150 W/m2 endpoint is supported by a few known concepts or prototype machines designed for the distributed wind space. Additionally, there is an increased incentive to go to lower specific power in the lower wind quality locations that distributed wind often finds itself (i.e., a different design optimum). It may also be the case that some existing distributed wind turbine platforms have more robust design margins in other portions of the turbine (e.g., the turbine mainframe) as a function of more limited means to have unique custom components that could further facilitate a move to lower specific power. In addition, improvements to balance-of-system costs (site preparation, wind turbine foundation, electrical, and tower) are also considered.
We assume performance converges over time as technology advancements are adopted within and across each of the respective size classes. This assumption is grounded in the absence of technical constraints that would preclude convergence. As a simplifying assumption, capacity factor improvements are equivalent across all modeled scenarios and analysis that
includes performance improvements.
To characterize the temporal rate of change in performance, we assume improvements will be fairly sizable in the near term and that innovation will have diminishing returns over time such that by 2050 continued annual improvements in capacity factor will be well below 1% per year. Resulting capacity factors are projected to 2050 by turbine size class and based on the methods described earlier (Lantz et al., 2016).
References
The following references are specific to this page; for all references in this ATB, see References.