Residential Battery Storage
The battery storage technologies do not calculate LCOE or LCOS, so do not use financial assumptions. Therefore all parameters are the same for the R&D and Markets & Policies Financials cases.
The 2023 ATB represents cost and performance for battery storage with a representative system: a 5-kW/12.5-kWh (2.5-hour) system. It represents only lithium-ion batteries (LIBs) - those with nickel manganese cobalt (NMC) and lithium iron phosphate (LFP) chemistries - at this time, with LFP becoming the primary chemistry for stationary storage starting in 2021. There are a variety of other commercial and emerging energy storage technologies; as costs are characterized to the same degree as LIBs, they will be added to future editions of the ATB.
The National Renewable Energy Laboratory's (NREL's) Storage Futures Study examined energy storage costs broadly and specifically the cost and performance of LIBs (Augustine and Blair, 2021). This report is the basis of the costs presented here (and for distributed commercial storage and utility-scale storage); it incorporates base year battery costs and breakdown from (Ramasamy et al., 2022) that works from a bottom-up cost model. The bottom-up battery energy storage systems (BESS) model accounts for major components, including the LIB pack, inverter, and the balance of system (BOS) needed for the installation. However, we note though that, during the time elapsed between the calculations for the Storage Futures Study and the ATB release, updated values were calculated as more underlying data were collected. Though these changes are small, we recommend using the ATB data rather than those published with the Storage Futures Study.
2021 costs for residential BESS are based on NREL’s bottom-up BESS cost model using the data and methodology of (Ramasamy et al., 2022), who estimated costs for only AC coupled systems. We use the same model and methodology, but we do not restrict the power or energy capacity of the BESS to two options. Key modeling assumptions and inputs are shown in Table 1. We assume 2022 battery pack costs of $283/kWhDC 2021 USD (Ramasamy et al., 2022).
Model Component | Modeled Value | Description |
System size | 5 kW power capacity 2.5 E/P ratio | Battery capacity is in kWDC. E/P is battery energy to power ratio and is synonymous with storage duration in hours. |
Battery pack cost | $283/kWh | Battery pack only (BNEF, 2021) |
Battery-based inverter cost | $183/kWh | Assumes a bidirectional inverter (BNEF, 2020), converted from $/kWh for 5 kW/12.5 kWh system |
Supply chain costs | 6.5% (U.S. average) | Markup is estimated from cost of battery, battery inverter, and BOS. |
Installation labor cost | $34.7/hour for hardware installation and electrical work | Assumes U.S. average pricing |
Engineering fee | $95 per system design | Engineering design and professional engineer-stamped calculations and drawings |
Permitting, inspection, and interconnection | $1,633 including $286 permit fee per system | Includes commissioning and interconnection labor, and permitting fee |
Sales and marketing (customer acquisition) | $3,851 per system installation | Assume per closed sale, associated with selling a storage system versus selling a PV system. |
Overhead (general and administrative) | $2,285 per system installation | Rent, building, equipment, staff expenses are not directly tied to permitting, inspection, and interconnection; customer acquisition; or direct installation labor. |
Profit | 17% | A fixed percentage margin is applied to battery, battery inverter, BOS, installation labor, supply chain, and sales tax. |
As with utility-scale BESS, the cost of a residential BESS is a function of both the power capacity and the energy storage capacity of the system, and both must be considered when estimating system cost. Furthermore, the Distributed Generation Market Demand (dGen) model does not assume specific BESS system sizes, and it needs an algorithm to estimate residential BESS system cost based on the attributes of the residences (agents) it generates.
We develop an algorithm for stand-alone residential BESS cost as a function of power and energy storage capacity using the NREL bottom-up residential BESS cost model (Ramasamy et al., 2022) with some modifications.
Scenario Descriptions
Available cost data and projections are very limited for distributed battery storage. Therefore, the battery cost and performance projections in the 2023 ATB are based on the same literature review as that done for utility-scale and commercial battery cost projections: battery cost and performance projections in the 2023 ATB are based on a literature review of 14 sources published in 2021 or 2022, as described by Cole and Karmakar (Cole and Karmakar, 2023). Three projections for 2022 to 2050 are developed for scenario modeling based on this literature review.
In all three scenarios of the scenarios described below, costs of battery storage are anticipated to continue to decline. The Storage Futures Study (Augustine and Blair, 2021) describes that most of this cost reduction comes from the battery pack cost component with minimal cost reductions in BOS, installation, and other contributions to the cost. The Storage Futures Study report (Augustine and Blair, 2021) indicates NREL, BloombergNEF (BNEF), and others anticipate that the growth of the overall battery industry - across the consumer electronics sector, the transportation sector, and the electric utility sector - will lead to cost reductions. Additionally, BNEF and others indicate changes in lithium-ion chemistry (e.g., switching from cobalt) will also reduce cost. A third key factor is ongoing innovation with significant corporate and public research on batteries. Finally, the growth in the market (effective learning-by-doing) and more diversity of chemistries will expand and change the dynamics of the supply chain for batteries resulting in cheaper inputs to the battery pack (Mann et al., 2022).
The three scenarios for technology innovation are:
- Conservative Technology Innovation Scenario (Conservative Scenario): The conservative projection consists of the maximum projection in 2022, 2023, 2024, 2025, and 2030 among the 14 cost projections from the literature review (Cole and Karmakar, 2023). Defining the points in 2050 is more challenging because the projections with the least cost reduction only extend to 2030. The projection with the smallest relative cost decline after 2030 showed battery cost reductions of 5.8% from 2030 to 2050. This 5.8% is used from the 2030 point in defining the conservative cost projection. In other words, the battery costs in the Conservative Scenario are assumed to decline by 5.8% from 2030 to 2050.
- Moderate Technology Innovation Scenario (Moderate Scenario): The moderate projections are taken as the median point in 2022, 2023, 2024, 2025, 2030, and 2050 from the 14 projections reviewed. The projections that are consistent with the median in 2030 do extend through 2050, which is why the median projection is used for 2050.
- Advanced Technology Innovation Scenario (Advanced Scenario): The advanced projections are taken as the lowest cost point in 2022, 2023, 2024, 2025, 2030, and 2050 from the 14 projections reviewed. The lowest cost projections also extend through 2050, allowing the lowest cost projection to be used for 2050.
Scenario Assumptions
Scenario assumptions for residential BESS were derived using a literature review, and are not based on learning curves or deployment projections.
For a 5-kW, 12.5-kWh battery, the technology-innovation scenarios for residential BESS described above result in CAPEX reductions of 17% (Conservative Scenario), 30% (Moderate Scenario), and 52% (Advanced Scenario) between 2022 and 2035. The average annual reduction rates are 1.4% (Conservative Scenario), 2.3% (Moderate Scenario), and 4.0% (Advanced Scenario).
Between 2035 and 2050, the CAPEX reductions are 4% (0.3% per year average) for the Conservative Scenario, 22% (1.5% per year average) for the Moderate Scenario, and 31% (2.1% per year average) for the Advanced Scenario.
Methodology
NREL does not maintain future cost projections for residential BESS for the ATB as it does for utility-scale systems. Instead, we base residential BESS cost projections on the NREL bottom-up cost model for residential systems combined with component cost projections from BloombergNEF (BNEF). The cost model has published cost projections for a 5-kW/14-kWh BESS system through 2030 (BNEF, 2020), and the projections are based on learning rates and future capacity projections.
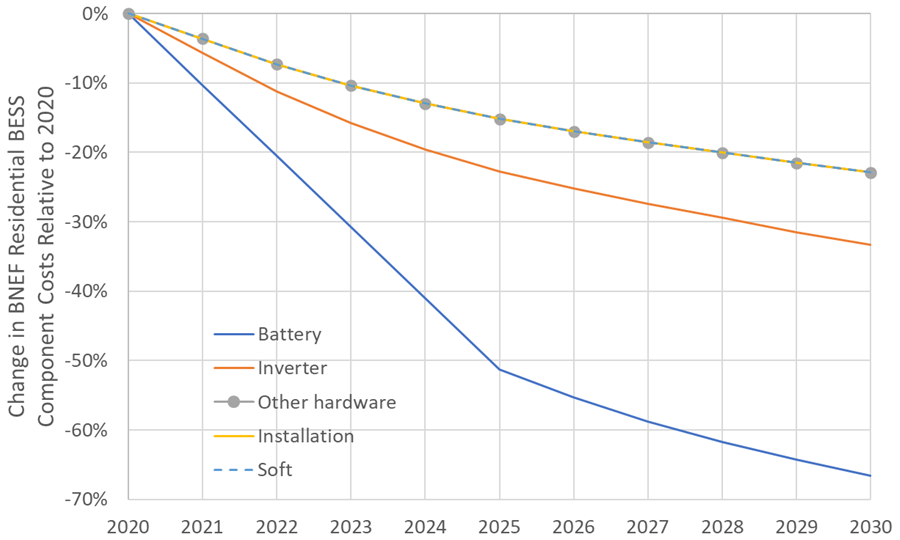
Data Source: (BNEF, 2019)
The methodology involves the following the steps to generate the Moderate Scenario future cost projections in detail:
- Estimate base year costs for a range of BESS power and energy capacity combinations using the NREL bottom-up residential BESS cost model.
- Record total and component cost results.
- Assign component costs to categories according to Table 1.
- Moderate Scenario: For each future year, apply cost reductions.
- Apply cost reductions from the BNEF projections (BNEF, 2020) to the corresponding component cost category for each BESS considered.
- BNEF projections only go to 2030. We assume residential BESS component costs decline by an additional 25% from 2030 to 2050, similar to the assumption used in the ATB utility-scale BESS cost projections in the 2022 ATB (Cole and Frazier, 2020).
- Advanced Scenario and Conservative Scenario: Apply cost projections from the corresponding ATB utility-scale BESS scenario to all component costs.
- Sum the component costs to get the total BESS cost in future years. For each future year, develop a linear correlation relating BESS costs to power and energy capacity:
- BESS cost (total $) = c1 * PB + c2 * EB + c3
- Where PB = battery power capacity (kW) and EB = battery energy storage capacity ($/kWh), and ci = constants specific to each future year.
Capital Expenditures (CAPEX)
Definition: The bottom-up cost model documented by (Ramasamy et al., 2022) contains detailed cost bins for both solar only, battery-only, and combined systems. Though the battery pack is a significant cost portion of the cost of the battery system, it is a fraction of the cost of the system. This cost breakdown is different if the battery is part of a hybrid system with solar PV or a stand-alone system. The total costs by component for residential-scale stand-alone battery are demonstrated in Figure 2 for two different examples of systems.
Current Year (2022): The Current Year (2022) cost estimate is taken from Ramasamy et al. (Ramasamy et al., 2022) and is currently in 2021 USD.
Within the ATB Data spreadsheet, costs are separated into energy and power cost estimates, which allows capital costs to be calculated for durations other than 4 hours according to the following equation:
$$\text{Total System Cost (\$/kW)} = \bigg[ \text{Battery Pack Cost (\$/kWh)} \times \text{Battery Energy Capacity (kWh)} \; +$$
$$ \text{Battery Power Capacity (kW)}\times \text{BOS Cost (\$/kW)} \; +$$
$$\text{Battery Power Constant (\$)} \bigg] / \; \text{Battery Power Capacity (kW)}$$
For more information on the power versus energy cost breakdown, see (Cole and Frazier, 2020). For items included in CAPEX, see the table below.
Future Projections: Future projections are based on the same literature review data that informed (Cole and Frazier, 2020), who generally used the median of published cost estimates to develop a Mid Technology Cost Scenario and the minimum values to develop a Low Technology Cost Scenario. However, as the battery pack cost is anticipated to fall more quickly than the other cost components (which is similar to the recent history of PV system costs), the battery pack cost reduction is taken from BNEF (BNEF, 2020) and is reduced more quickly. This tends to make the longer-duration batteries (e.g., 10 hours) decrease more quickly and shorter-duration batteries (e.g., 2 hours) decrease less quickly into the future. All durations trend toward a common trajectory as battery pack costs decrease into the future.
Operation and Maintenance (O&M) Costs
Base Year: (Cole and Karmakar, 2023) assume no variable O&M (VOM) costs. All operating costs are instead represented using fixed O&M (FOM) costs. The fixed O&M costs include battery augmentation costs, which enables the system to operate at its rated capacity throughout its 15-year lifetime. FOM costs are estimated at 2.5% of the capital costs in $/kW. Items included in O&M are shown in the table below.
Future Years: In the 2023 ATB, the FOM costs and VOM costs remain constant at the values listed above for all scenarios.
Capacity Factor
The cost and performance of the battery systems are based on an assumption of approximately one cycle per day. Therefore, a 4-hour device has an expected capacity factor of 16.7% (4/24 = 0.167), and a 2-hour device has an expected capacity factor of 8.3% (2/24 = 0.083). Degradation is a function of this usage rate of the model and systems might need to be replaced at some point during the analysis period. We use the capacity factor for a 4-hour device as the default value for ATB due to the expectation that 4-hour durations are more typical in the utility-scale market.
Round-Trip Efficiency
Round-trip efficiency is the ratio of useful energy output to useful energy input. (Cole and Karmakar, 2023) identified 85% as a representative round-trip efficiency, and the 2023 ATB adopts this value.
References
The following references are specific to this page; for all references in this ATB, see References.