Financial Cases and Methods
Financial assumptions impact the levelized cost of energy (LCOE) by changing the cost of capital needed to finance electricity generation projects. The equations and variables used to estimate LCOE are defined on the Equations and Variables page.
This section of the ATB focuses on the input variables to the weighted average cost of capital (WACC), which is used in the Electricity ATB as the discount rate input to the capital recovery factor (CRF) for the LCOE formula. The CRF and the project finance factor determine the fixed charge rate (FCR), which incorporates other elements of project finance including the:
- Combined federal and state tax rate
- Depreciation schedule of the asset
- Average inflation rate
- Tax incentives.
Because the elements mentioned above are fixed or would require changes to legislation, the discussion below focuses on the variables in WACC.
Financial Assumptions Cases
Two different cases with differing project finance assumptions are used within the ATB:
- Financing structure without tax incentives or changes from 2020: LCOE improvement is therefore based on R&D improvements alone (R&D Only Case).
- Market + Policies Financial Assumptions Case, in which there are market and policy changes (Market + Policies Case).
Use the following chart to explore the differences between the two financial assumptions cases.
To explore the effect of financial assumptions on LCOE, select a technology and financial assumptions case.
To explore the financial assumptions, select a financial parameter (interest rate, rate of return, CRF, debt fraction, and WACC), technology, and financial assumptions case.
The R&D Only Case, the Market + Policies Case, and the methodology and assumptions are as follows:
- R&D Only Case
- Estimates technology-specific debt interest rates, return on equity rates, and debt fraction to reflect technological risk perception but with consistent sources of capital and ownership across technologies (i.e., independent power producer, self-financed)
- Holds tax and inflation rates constant at assumed 2020 values: 21% federal tax rate, 6% state tax rate (though actual state tax rates vary), and 2.5% inflation rate
- Excludes effects of tax credits
- Market + Policies Case
- Retains the technology-specific return on equity rates developed in the R&D Only Case
- Increases debt interest rates 1% between 2025 and 2030 for all technologies, reflecting an assumed return to long-term costs for underlying interest rates
- Assumes that average the inflation rate over the life of the project is 2.5%
- Applies federal tax credits and expires it as consistent with existing law and guidelines
- General Methodology and Assumptions
- Renewable Generators: Projects with financing terms are owned by independent power producers (IPPs) that have entered into long-term, fixed-price, take-or-pay power purchase agreements (PPAs) for the sale of the electricity. Though PPAs are not the only possible arrangement, they are the dominant form of asset ownership and electricity offtake for new renewable energy assets in the United States.
- Financing Structure: IPP structure is assumed to internally capture the full benefits of tax credits without the need for tax equity partnerships. Tax equity arrangements are currently a popular financing technique and generally offer lower-cost equity than sponsor equity, for a portion of the cost of eligible projects in exchange for the associated tax benefits, as not all companies can use all the tax benefits. However, they also come with the costs of structuring, arranging complex financial arrangements, and removing them when they are no longer needed.
- Conventional Generators: Financing terms reflect natural gas electric generation projects also owned by IPPs that sell power through either a short-term contract or a wholesale spot electricity market, which are otherwise known as "quasi-merchant" projects. Natural gas plants in unregulated markets have not historically entered electricity price contracts of the same length as renewable energy generation assets for a variety of reasons, including the challenges of fuel price hedging or contracting for more than a few years. Natural gas plants represent most of all recently installed conventional electricity generation, and they therefore represent "typical" financial transactions and financing terms.
- Risk Assessment: Financial assumptions reflect the differentiation of risk across technologies through varied financing terms. Although we recognize that in practice these risks may also be reflected in CAPEX, in soft costs, in a contingency fund, or elsewhere, we believe that reflecting risk in the financing terms provides the clearest and most consistent approach. Therefore, we use technology-specific debt interest rates, returns on equity, and debt fractions as a percentage of the total project to reflect technological risk perception. Debt fraction is calculated using a separate financial model, with technology-specific ATB inputs, based on technology-specific assumed debt service coverage ratios (DSCRs).
- Domestic Focus: Assumptions primarily reflect technology risk within the U.S. market, but some consideration is given to international development. An individual technology may receive more-favorable financing terms outside the United States as a result of different macroeconomic factors (e.g., different regulations or different interest rates), more government interventions or more market guarantees, or better market perception of technologies.
- Constant Technology Risk: Assumptions reflect no change in underlying risk perception for each technology over time. The modeled renewable technologies have globally deployed billions of dollars in CAPEX and currently receive more-favorable financing terms than other electric generation technologies. Though there is still opportunity for further risk perception reduction, because the cost of capital is so favorable, we assume a reduction in risk would likely result in higher leverage, providing equity investors with higher returns (which might have a neutral impact on the overall cost of capital) or electricity offtake agreements that are currently considered riskier than a long-term PPA (i.e., selling electricity into wholesale markets). It should be noted that even if the reduction of risk does not lower cost, it could increase adoption by opening more markets and opportunities. At the same time, the differences in risk between technologies reflect underlying technology risks of construction and operation.
- Cost Recovery Period: Both cases assume a constant cost recovery period—or period over which the initial capital investment is recovered—of 30 years for all technologies. The ATB also provides an option to look at cost recovery over a 20-year period.
Methods for Developing Financial Assumptions
This approach to generating the financial assumptions includes technology-specific financial assumptions to capture more granularity of current and future energy markets as well as to show changes in financing rates that are attributable to the reduction or elimination of tax credits for eligible technologies (see below for schedule reductions). Each technology has its own specific risk factors that might influence the underlying cost of financing. However, a multitude of other factors determine a project's cash flow risk, including:
- Political risk
- Regulatory uncertainty
- Project development risk
- Government support (if any)
- Ownership risk (IPP versus investor-owned utility versus public utility)
- Credit-worthiness of the electricity off-taker and the length of the contract (if any)
- Whether the electricity price is firm or changes with the market
- Supply and demand of competing electricity and sources of financing
- Underlying inflation rate, and the cost of the base rate (e.g., London Inter-Bank Offered Rate, or LIBOR).
Also, some projects might receive more-favorable financing because of economies of scale (or, for some investment sources, minimum investment thresholds). For these reasons, there is a wide range in financing costs across the United States, and the world, even for the same technology.
To reduce the impact on non-technology-based risk factors and create a consistent financial landscape across technologies, we assume financing terms for renewable energy projects owned by IPPs who enter into long-term PPAs. Though this is not the only possible arrangement, it is the dominant form of asset ownership and electricity offtake for new renewable energy assets in the United States. Also, PPAs avoid many of the financial risks associated with electricity offtake and price uncertainty. Financing terms for conventional energy generation reflect natural gas electric generation projects owned by IPPs that sell power through short-term contracts or through a wholesale spot electricity market—otherwise known as "quasi-merchant" projects.
Natural gas plants in unregulated markets have not historically entered electricity price contracts of the same length as renewable energy generation assets for a variety of reasons, including the challenges of fuel price hedging or contracting for more than a few years. Natural gas plants represent most of all recently installed conventional electricity generation and therefore most all conventional electricity generation financing, and financing terms. We collect data from a variety of sources that have exposure to different renewable energy technology financings, both in the United States and abroad. In doing so, we try to accurately represent typical financing costs for each technology as well as the differences, if any, between technologies. We collect data points for the:
- After-tax cost of levered equity during the construction and operation of the asset
- Cost of debt during the construction and operation of the asset
- Amount of debt provided during construction
- Required DSCR debt providers use to determine the amount of debt (i.e., leverage) they would provide a project during the operation of the asset.
In the 2020 ATB through the 2022 ATB—unlike the 2019 ATB and earlier editions—we add the cost of equity during construction. We make this change for two reasons:
- Construction debt providers almost always require a certain percentage of construction costs (typically the first dollars spent) to come from equity providers
- We hope to differentiate the cost of construction financing from the cost of financing during operations.
In particular, in ATB editions before 2020, the cost of equity for geothermal plants was assumed to be much higher than other technologies to account for the risks during construction. By separating these two financing periods, we can lower the cost of equity for a geothermal plant during operation to bring it more in-line with real-world financing terms.
The following figure summarizes data gathered for the cost of equity by technology type, and to a lesser extent, contract type. The orange box shows the ATB after-tax cost of equity assumptions during project operation. These values are based on data for the levered after-tax returns and are influenced by the difference in unlevered returns between technologies, as well as tax equity returns. As shown, utility-scale PV requires the lowest rates of return, followed by distributed PV and land-based wind, and finally, the remainder of renewable energy technologies. In general, the rates of return increase with an increase in operation risk (e.g., more resource uncertainty and higher chance of mechanical failure). Also shown is the premium charged for contracts with less electricity price certainty (e.g., corporate offtakers and hedges). Corporate offtakers may have lower credit ratings (i.e., more perceived risk of default) than regulated utilities, and hedges do not necessarily offer full coverage and may be for shorter durations than standard contracts provided by utilities. As shown in the figure , utility-scale PV requires the lowest after-tax levered rate of return, with a median of 7.75%, and it is followed by land-based wind at 9.00%. Given the limited data points for the other technologies (e.g., offshore wind, concentrating solar power [CSP], geothermal, hydropower, and natural gas), their overlapping data points, and the premium assessed over wind and PV by data sources that provided multiple values, we assume a 100 basis point premium over land-based wind. This premium is slightly lower than the premium reported by individual data sources when comparing these technologies to land-based wind (which range from 120 to 150 basis points); however, the 100 basis point premium provides values more consistent with their median data points. We were unable to obtain specific returns for distributed PV, but (Norton Rose Fulbright, 2020) estimates that tax equity rates for distributed PV are approximately 100 basis points higher than utility-scale PV transactions; therefore, we assume a similar premium for after-tax levered equity returns.
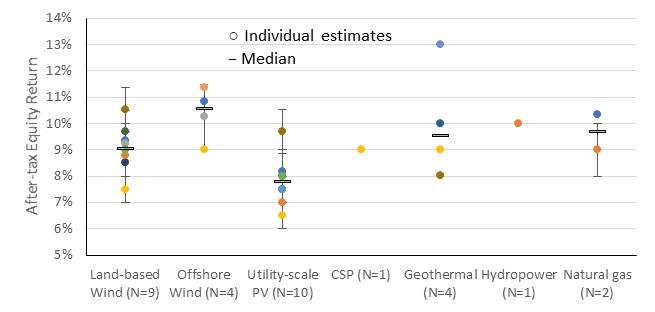
Sources: (Dumoulin-Smith et al., 2020); (BNEF, 2019); (Nextera Energy, 2019); (Ørsted, 2018); (Ormat Technologies, 2020); (Norton Rose Fulbright, 2020); and National Renewable Energy Laboratory (NREL) telephonic or electronic mail interviews with 10 unnamed financiers on January 22, 2020; January 29, 2020; February 11, 2020; May 13, 2020; May 14, 2020; and May 18, 2020
Based on industry interviews, we include a 2% premium on the cost of equity during construction for each project, relative to the cost of equity during plant operation, to account for construction risk, with the exception of geothermal, coal, and natural gas. Coal and natural gas do not assume a construction cost premium, but they have a leverage rate of 55%, consistent with the assumptions in (Theis, 2021). Additionally, we separate the cost of equity during construction for geothermal into two stages: predrilling (assumed to be 15%) and post-drilling (with assumed site control in place, permitting completed, and a PPA contracted), with no premium on the cost of equity relative to the cost of equity during plant operation.
The following figure shows collected data on the cost of debt for renewable energy assets. Publicly available data points represent a mix of renewable energy technologies, project and portfolio financing's, timing and loan tenors (i.e., length) and actual versus modeled rates. Though "like-to-like" data comparisons are ideal, the data are limited and the data collected show a fairly consistent and fairly narrow spread between technologies. For ATB modeling purposes, we assume a 4.0% term interest rate (inclusive of fees and reserves) for all renewable energy technologies, reflecting the mix of data types and level of precision of in data.
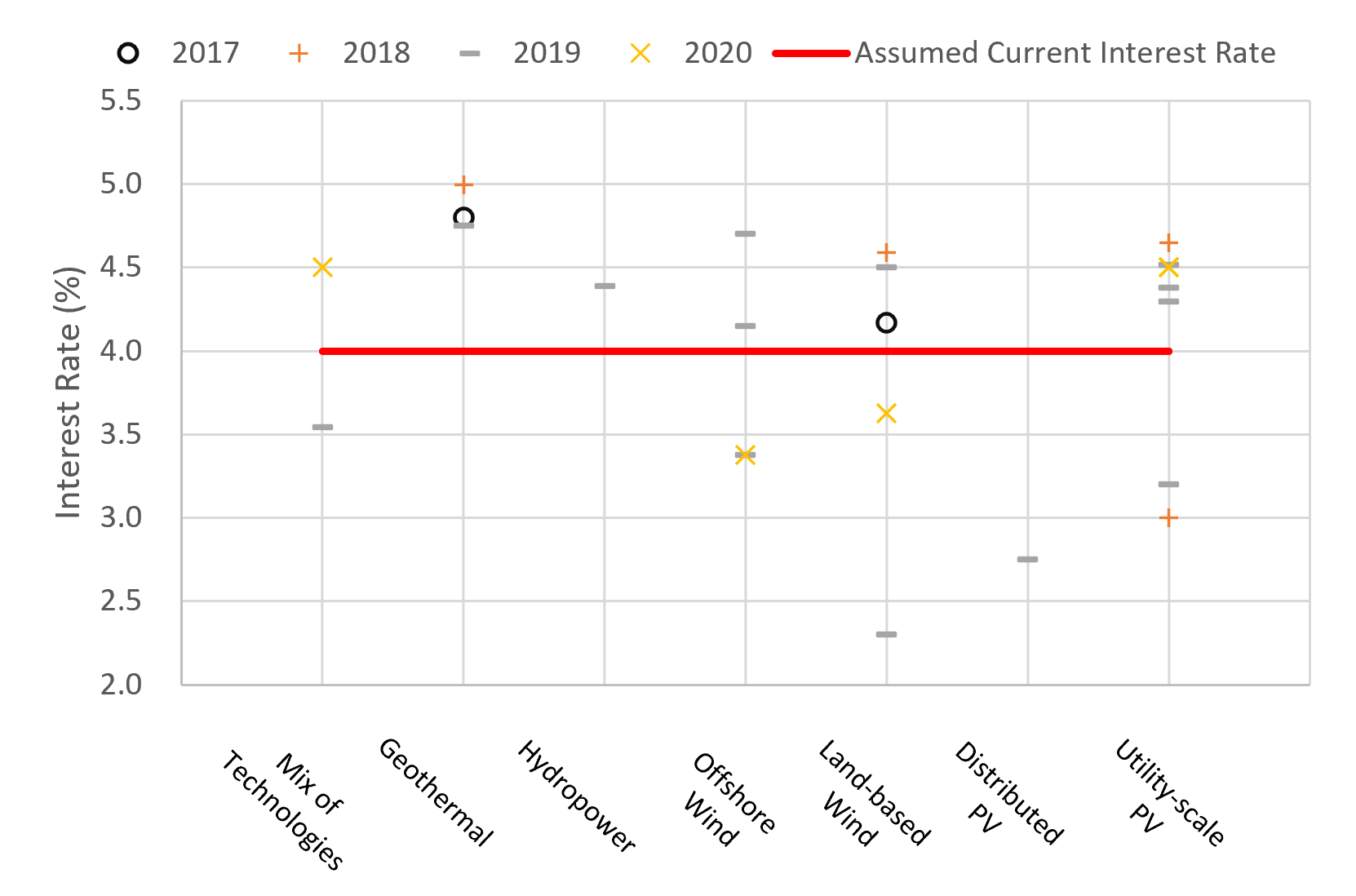
Sources: (Berkshire Hathaway Energy, 2018), (Terraform Power, 2019), (Ormat Technologies, 2020), (Brookfield Renewable Partners, 2020), (Dumoulin-Smith et al., 2020), (Guillet and Green Giraffe, 2018), (Norton Rose Fulbright, 2020), (Norton Rose Fulbright, 2019b), (Norton Rose Fulbright, 2019a), (Ormat Technologies, 2020), (Ormat Technologies, 2019), and (Ormat Technologies, 2018)
According to Norton Rose Fulbright (Norton Rose Fulbright, 2020), interest during construction is approximately 0.5% lower than term debt, which we implement for all technologies except offshore wind, geothermal, and hydropower, where there is inherently greater construction risk. Additionally, in the Market + Policies Case, we assume interest rates increase from 2025 to 2030 by 1% (i.e., 100 basis points) across the board, to account for the recent lower interest rate environment. Though the expectation that interest rates will rise in the future is already priced in the swap rate (interest rate swaps represent the trading of floating interest rates for fixed interest rates and are a standard part of renewable energy debt financing) of recent debt pricing, a return to the mean would likely increase rates more. We choose 2025 as the starting point for the interest rate increase given the current uncertainty about interest rates.
The following figure shows collected data on the DSCRs for renewable energy assets and the assumed DSCR for the 2022 ATB analysis. P50 represents an average-level of energy production (or 50% likelihood to generate that much or more electricity), while P99 represents a production level that the project has a 99% chance of exceeding. The assumed P50 DSCR, per technology, is based on collected data of P50 DSCR and is influenced by the difference in average DSCRs between technologies in P90 and P99 scenarios, as well as collected DSCRs from ratings agencies for stress modeling cases (not shown).
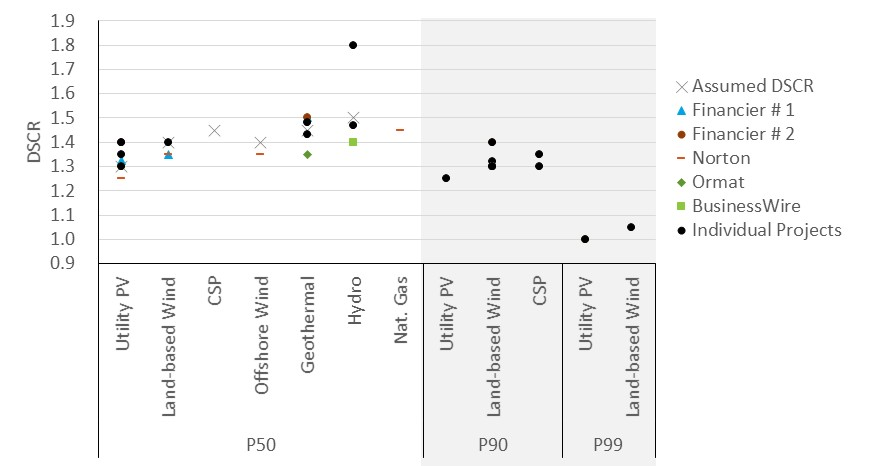
Sources: (Norton Rose Fulbright, 2019a), (Norton Rose Fulbright, 2020), (Ormat Technologies, 2020), (Business Wire, 2015)(Credit Agricole Securities, 2018a), (Credit Agricole Securities, 2018b), (Business Wire, 2015), and NREL telephonic interviews with two unnamed financiers on January 31, 2020 and March 20, 2020
Similar to the cost of equity, technologies with perceived greater operating risk from the financial community tend to have higher DSCRs. This is validated by a recent report from Fitch Ratings, which stated that, "More than a decade of analysis shows that solar resources are consistently more stable and predictable than wind, resulting in less volatile revenues and generally higher ratings" (Crowell, 2020). As another example, land-based and offshore wind are reported to have the same DSCR, because the greater O&M uncertainty of offshore wind (i.e., maintaining an asset in the ocean) was counterbalanced by having more certainty in wind speed than land-based wind.
The following table summarizes financial assumptions by technology during the project's operation.
Operation | Construction | ||||||
---|---|---|---|---|---|---|---|
Technology | Electricity Sales | After-Tax Equity Returns | Interest Rate of Term Debt in 2019/2030 | DSCR | After-Tax Equity Returns | Interest Rate of Construction Debt in 2020/2030 | Leverage |
Utility PV and utility PV+battery | PPA | 7.75% | 4.0%/5.0 | 1.30 | 9.5% | 3.5%/4.5% | 80% |
Residential and commercial PV | PPA | 8.75% | 4.0%/5.0 | 1.30 | 10.5% | 3.5%/4.5% | 80% |
CSP | PPA | 10.0% | 4.0%/5.0 | 1.45 | 12.0% | 3.5%/4.5% | 80% |
Land-based wind | PPA | 9.0% | 4.0%/5.0 | 1.40 | 11.0% | 3.5%/4.5% | 80% |
Offshore wind | PPA | 10.0% | 4.0%/5.0 | 1.40 | 12.0% | 4.0%/5.0 | 80% |
Distributed wind | PPA | 9.0% | 4.0%/5.0 | 1.40 | 11.0% | 3.5%/4.5% | 80% |
Geothermal | PPA | 10.0% | 4.0%/5.0 | 1.45 | Predrilling: 15% Post-drilling: 10% | 4.0%/5.0 | Predrilling: 0% Post-drilling: 75% |
Hydropower | PPA | 10.0% | 4.0%/5.0 | 1.50 | 12.0% | 4.0%/5.0 | 80% |
Pumped storage hydropower | PPA | 10.0% | 4.0%/5.0 | 1.50 | 12.0% | 4.0%/5.0 | 80% |
Natural gas | Quasi-merchant | 10.0% | 5.0%/6.0 | 1.45 | 10.0% | 5.0%/5.0% | 55% |
Another important factor in determining the financial structure for a renewable energy project is the level of tax credits, if any. Below is a summary of the tax credits assumed in analysis for the 2022 ATB. We assume eligible projects will use the full duration of the "safe harbor" window in order to receive the maximum tax credit level, which is 4 years for onshore geothermal, hydropower, solar, and onshore wind projects, and 10 years for offshore wind. We also assume offshore wind elects the investment tax credit (ITC) over the production tax credit (PTC) to maximize economic value. Finally, we assume geothermal elects the PTC over the permanent 10% ITC from 2020 through 2026 in order to maximize economic value; from 2027 onwards, when the PTC is no longer available, geothermal uses the ITC.
We never assume a 40% PTC (or similar ITC percentage for offshore wind) because of the step back up to 60% in the following year. Hydropower facilities that are eligible to receive the PTC include new power dams, among others.
Based on these assumptions, as well as capital expenditures (CAPEX), fixed operation and maintenance (FOM), variable operation and maintenance (VOM), capacity factor, and fuel costs, we run financial models to calculate a technology's leverage as governed by the minimum DSCR, assuming a debt amortization schedule of 18 years (which is a common debt amortization period for U.S. renewable energy projects, even if the term of debt is shorter). In general, leverage only varies significantly over time due to changes to interest rate, tax rate, and the amount of tax credits received, but because of CAPEX, FOM or capacity factor. For this reason, the same leverage can be used by a technology for different resource areas. The exception to this is projects receiving the PTC (i.e., land-based wind); because PTC value represents a different percentage of project costs for different resource classes, leverage changes. A similar, but smaller, change can also be seen for natural gas, depending on fuel price. Because we cannot input different leverage values for different resource classes in the ATB (because of current programming constraints), we use the leverage for Wind Speed Class 4 , because it is currently the most common resource level for land-based wind systems. It is important to keep in mind that these relationships occur because the financial model (and the ATB) solve for LCOE, which represents the electricity rate needed to achieve specific economic returns. If the financial models were to solve for economic returns, given an assumed electricity rate, the leverage on projects with higher capacity factors (all other things being equal) would be greater than those with lower capacity factors. See the parameter value summary above for the calculated leverages for the ATB.
In the R&D Only cases, leverage between renewable energy technologies only varies from 66% to 76%. A certain degree of variability in leverage exists between technologies because of differences in capacity factors, return on equity, DSCR, and interest rates. In the Market + Policies Case, the leverage on technologies with tax credits increases as these credits phase out, and then decreases incrementally from 2025 to 2030 as interest rates rise. Solar and geothermal technologies, in the Market + Policies Case, have the lowest leverage in 2030 because of the continued use of the 10% ITC. Using these values, we calculated WACC for the different technologies, which is summarized in the parameter value summary above.
In the R&D Only Case, the after-tax WACC varies from 4.2% to 5.3% for renewable energy technologies, and it is 5.4% for natural gas. A certain degree of variability in WACC exists because of underlying construction and operation risks, as well as contract risk for natural gas. In the Market + Policies Case, the WACC on technologies with tax credits decreases as these credits phase out. WACC subsequently increases from 2025 to 2030 as interest rates rise.
It is important to remember that financing costs are one piece of the overall cost competitiveness of a project. Though projects receiving tax credits might have lower leverage, and thus a higher WACC, they benefit from the tax credits, which overall, reduce the LCOE. Likewise, Wind Speed Class 1 would have a higher WACC than Wind Speed Class 10, but that is because the cost of energy of Wind Speed Class 10 is much higher, and so can support a higher modeled debt fraction. Natural gas has the highest calculated leverage, but it still has the highest WACC because of the increased cost of debt and equity (in part because it is the only technology analyzed in the ATB that does not have fully contracted cash flows). However, in 2019, natural gas still has some of the lowest ATB LCOEs, does not depend on long-term fixed contracts, and might be more competitive in markets for reasons not captured by LCOE (e.g., capacity credit).
Looking forward, we do not assume a change to the cost of equity or DSCR because many factors might influence these variables. For example, political, corporate, or regulatory changes may push the cost of equity and DSCR higher or lower (e.g., impact the ability to get long-term contracted cash flows). The renewable energy industry is actively working to continue to remove the risk and uncertainty associated with these technologies and to provide more-consistent expectations of electricity production (e.g., lower failure rates, better energy production forecasting, and more consistent resource availability through higher wind towers). However, renewable energy assets already receive favorable financing because of their ability to receive long-term fixed electricity contracts, the relative demand for "green investments," and the lower-risk benefits of tax credits. Which of these factors will change in the future is unclear. Utilities might stop offering long-term contracts or project owners might be exposed to more curtailment risk. On the other hand, with many states pushing for more carbon-free electricity, utilities might see these long-term contracts as low-cost options to satisfying these requirements; without long-term contracts, utilities might incur higher costs because of the increase in financing costs caused by shorter contracts.
Additionally, while curtailment and value loss could be more of an issue in the future as renewable energy assets make up a larger share of the total electricity generation mix, many active mitigation strategies are being developed, both by individual actors (e.g., pairing storage with renewable energy electric generation) or through larger, grid-level activities. And, while improvements in DSCR, through lower risk perception, might support higher project leverage, this benefit might be counteracted by higher equity returns achieved through higher leverage. Historically, many international renewable energy projects have had slightly higher leverage and higher equity returns. For the 2022 ATB, we collect data from 174 global renewable energy projects.
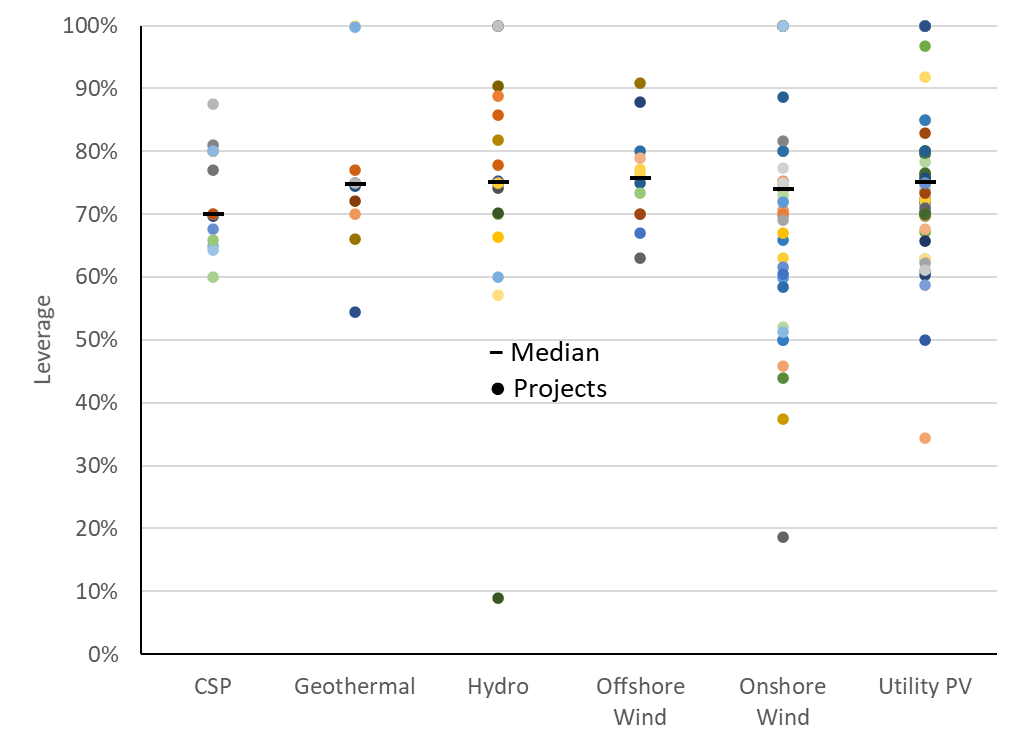
Sources: (BNEF, forthcoming), (World Bank, 2014), (New Energy Update, 2019), (Thompson Reuters, 2018), (Thompson Reuters, 2019), and an NREL personal communication, 2020
Although leverage varies dramatically among individual projects, the median leverage is generally around 75%, with no clear differences between technologies. And the differences are lessened when removing the projects that receive more than 80% financing through debt, with the median dropping to around 70% for all technologies, which roughly matches the approximate leverage calculated for technologies in the 2022 ATB. Many of these highly leveraged projects received government, or quasi-government (e.g., World Bank), assistance in the form of nonmarket loan terms (e.g., 30-year terms), loan guarantees, or favorable credit guidelines. Many also received higher returns than projects in the United States, in the mid-teens, which allowed for even greater leverage, as higher equity returns are achieved by higher-priced electricity or other revenue streams, which allow more debt for the same DSCR. This is not to say that research on risk reduction is not a critical part of providing favorable renewable energy project economics but only that reductions in perceived risk will not necessarily lower the absolute cost of financing from current levels—rather it will likely keep rates as low as the market will bear. Reducing risk also allows for increased deployment by opening markets or projects that would have otherwise been too risky.
References
The following references are specific to this page; for all references in this ATB, see References.