Geothermal
2024 Annual Technology Baseline (ATB) data for geothermal are shown above. The Base Year hydrothermal costs are derived from data from actual geothermal power plants. Near-term enhanced geothermal system (EGS) costs are predictions based on reported improvements in a package of technologies currently being field demonstrated. Hence, they have no calibration to actual project deployment because there are no commercial-scale dedicated EGS plants in operation in the United States. The future cost projections primarily use bottom-up models derived from the analysis and results of the GeoVision: Harnessing the Heat Beneath our Feet report with significant technology and cost assumption updates reflected in the 2023 Enhanced Shot Analysis (DOE, 2019); (Augustine et al., 2023). The GeoVision analysis is a collaborative multiyear effort with contributors from industry, academia, national laboratories, and federal agencies. The GeoVision report updated resource potential estimates as well as recently published and projected capital and operation and maintenance (O&M) costs through evaluation of recent industry trends. It also predicted advancements in areas such as drilling efficiency and materials and EGS stimulation success. Anticipated drilling and EGS improvements enable reduced development timelines, CAPEX, and financing rates (DOE, 2019); (Augustine et al., 2023); (Schulz and Livescu, 2023); (El-Sadi et al., 2024); (Dupriest and Noynaert, 2024); (DOE, 2024).
The 2021 U.S. Geothermal Power Production and District Heating Market Report published by the National Renewable Energy Laboratory (NREL) confirms many of the industry trends reported in GeoVision (Robins et al., 2021). For example, the Base Year values of levelized cost of energy (LCOE) for hydrothermal plants fall within the bounds of other publications and recent geothermal power purchase agreements (PPAs). On the EGS front, significant technical progress continues since the publication of the 2021 Market Report continues to be made through the Frontier Observatory for Research in Geothermal Energy (FORGE) initiative, funded by the U.S. Department of Energy (DOE), and other ongoing industry-led pilot demonstrations and commercial projects. With the addition of new EGS research and demonstration (e.g., the EGS Pilot Demonstration) activities under the Bipartisan Infrastructure Law Enhanced Geothermal Systems (EGS) Pilot Demonstrations funding opportunity as well as the Enhanced Geothermal Shot initiative launched in 2023, EGS technical progress toward economical operations is accelerating. Technical progress in drilling and reservoir stimulation is already being reported with exponential intra- and interproject learnings (Norbeck et al., 2023); (El-Sadi et al., 2024); (Dupriest and Noynaert, 2024). There has also been an uptick in private sector investment in EGS development. Between 2021 and early 2014, about $416 million has been invested in companies leading the development and commercial deployment of EGS (DOE, 2024). These efforts have led to recent signings of PPAs between EGS developers and clean energy offtakers for electricity from EGS-based power plants with some scheduled to begin power generation by 2026 (DOE, 2024). The increase in commercial readiness, alongside reported progress in EGS field demonstrations, has informed significant updates to the baseline performance assumptions and plant capacities in the 2024 ATB. The terms for public geothermal PPAs signed from November 2019 through June 2023 can be found in the table below (Robins et al., 2021); (DOE, 2024).
Project* | State | Size (megawatts [MW]) | Pricing ($/megawatt-hour [MWh]) | Term (years) |
---|---|---|---|---|
Hell’s Kitchen | California | 40 | 75 | 25 |
Whitegrass | Nevada | 3 | 67.50 | 25 |
Star Peak | Nevada | 12.5 | 70.25 | 25 |
Casa Diablo | California | 16 | 68 | 20 |
Puna | Hawaii | 46 | 70 | 30 |
Makushin | Alaska | 30 | 69 | 30 |
Geysers - Sacramento | California | 100 | 99 | 10 |
Geysers - Oakland | California | 2 | 70 | 12 |
* Only PPAs with pricing information are included. Other signed PPAs with undisclosed pricing information can be found in (Robins et al., 2021); (DOE, 2024).
The three scenarios for technology innovation are as follows:
- Conservative Technology Innovation Scenario (Conservative Scenario): Continuation of current industry trends in drilling (e.g., minor efficiency improvements with little to no increase in rate of penetration [ROP]) and EGS (e.g., limited increase in flow rate and stimulation success rate) result in minor CAPEX improvements by 2035.
- Moderate Technology Innovation Scenario (Moderate Scenario): Drilling advancements (e.g., doubled ROP and bit life from GeoVision baseline and reduced number of casing intervals and associated drilling materials) detailed as part of the GeoVision report (DOE, 2019) and EGS stimulation successes from DOE-funded EGS Collab and FORGE projects (Kneafsey et al., 2022); (Dupriest and Noynaert, 2024) and industry demonstration projects (Norbeck et al., 2023); (El-Sadi et al., 2024); (So et al., 2024) result in cost improvements that are fully achieved industrywide by 2035. Also, as part of 2024 ATB updates, this scenario assumes EGS power plants are built to a capacity of 40 megawatts (MW).
- Advanced Technology Innovation Scenario (Advanced Scenario): Substantial drilling and EGS advancements (e.g., significantly increased ROP, bit life, and EGS stimulation success; limited casing intervals; significantly reduced consumption of drilling materials; and reduced development timelines) as modeled in the Technology Improvement scenario of the GeoVision report result in cost improvements achieved by 2035. Advanced Scenario EGS power plants are assumed to be built with 100 MW of capacity to maximize project efficiency. In addition, permitting timelines reflect anticipated permit streamlining effects of a National Renewable Energy Coordination Office, as enacted through the Energy Act of 2020.
Resource Categorization
Within the 2024 ATB, geothermal resources broadly consist of two main types: hydrothermal and EGS. Although nascent technologies such as closed-loop and superhot rock systems fall within the category of geothermal for electricity generation, they are not covered in the 2024 ATB. Hydrothermal systems are naturally occurring zones of earth-heated circulating fluid that can be exploited for electricity generation if certain minimum temperatures and flow rates are achieved for a given power plant technology. Hot rocks suitable for EGS exhibit naturally occurring zones of heat but lack sufficient fluid flow and require substantial stimulation to enhance permeability. These two resource types are subdivided based on site-specific resource characteristics and compatible power plant technology into two types of energy conversion processes used to generate geothermal electricity:
- Binary plants use a heat exchanger and secondary working fluid with a boiling point below that of water to transfer geothermal energy through an organic Rankine cycle. Although commercial cycles can operate up to a maximum temperature of 300°C, in the geothermal ATB, they generally apply to lower-temperature systems (<200°C) because of the current maximum operating temperature of pumping technology. Generally, binary plants have higher CAPEX than flash plants because of the increased number of components, their lower-temperature operation, and a general requirement that several wells be drilled for a given power output.
- Flash plants generate steam through a pressure change of the thermal fluid that directly drives a turbine. This technology generally applies to higher-temperature systems. Because of the reduced number of components and higher-temperature operation, these systems generally produce more power per well, requiring fewer wells and reducing drilling costs. These systems generally have lower CAPEX than binary systems.
Hybrid plants, or a combination of binary and flash systems, are used in some locations but are not modeled in the ATB.
The ATB defines flash resources as those with temperatures at or above 200°C and binary resources as those with temperatures from 110 to <200°C. EGS resources are further subdivided into near-hydrothermal field EGS (NF-EGS) and deep EGS. The resulting geothermal resource supply curves consist of six categories: hydrothermal flash, hydrothermal binary, NF-EGS flash, NF-EGS binary, deep-EGS flash, and deep-EGS binary. For illustration in the ATB, six representative geothermal plants are shown with design parameters based on each resource category. Based on the technology definitions for the 2024 ATB, the hydrothermal technologies are considered mature technologies whereas the NF-EGS and deep-EGS technologies are considered nascent because there were no commercial EGS plants in operation or under construction in the Base Year (i.e., 2022).
Examples using these plant types in each of the three resource categories (hydrothermal, NF-EGS, and deep EGS) are shown in the ATB visualization.
Technology | Temperature (°C) | >=200 | 150–200 | 135–150 | <135 |
---|---|---|---|---|---|
Hydrothermal | Number of identified sites | 20 | 21 | 17 | 57 |
Total identified capacity (MW) | 3,824 | 783 | 378 | 581 | |
Average overnight capital cost, or OCC ($/kW) | 4,350 | 9,483 | 10,196 | 18,956 | |
Min OCC ($/kW) | 3,091 | 4,882 | 8,185 | 12,685 | |
Max OCC ($/kW) | 5,922 | 38,108 | 12,209 | 26,440 | |
Example of plant OCC ($/kW)* | 4,759 | 6,349 | N/A | ||
Number of undiscovered sites | 7 | 8 | 2 | 17 | |
Total undiscovered capacity (MW) | 14,909 | 3,810 | 869 | 6,223 | |
Average OCC ($/kilowatt [kW]) | 3,738 | 7,425 | 10,098 | 19,058 | |
Min OCC ($/kW) | 3,307 | 6,759 | 10,098 | 15,157 | |
Max OCC ($/kW) | 4,412 | 8,298 | 10,098 | 23,885 | |
NF-EGS | Number of sites | 12 | 20 | N/A | |
Total capacity (MW) | 787 | 707 | |||
Average OCC ($/kW) | 5,469 | 10,395 | |||
Min OCC ($/kW) | 4,542 | 7,877 | |||
Max OCC ($/kW) | 7,161 | 13,456 | |||
Example of plant OCC ($/kW) | 6,535 | 9,140 | |||
Deep EGS (3–7 kilometers [km]) | Number of sites | N/A | N/A | N/A | |
Total capacity (MW) | 100,000+ | ||||
Average OCC ($/kW) | 12,396 | 22,133 | |||
Min OCC ($/kW) | 9,228 | 13,378 | |||
Max OCC ($/kW) | 17,948 | 33,512 | |||
Example of plant OCC ($/kW) | 7,630 | 13,508 |
OCC: overnight capital cost
* Same for both identified and undiscovered hydrothermal resources.
The hydrothermal geothermal resource potential is concentrated in the western United States. The total mean potential estimated by the U.S. Geological Survey (USGS) in 2008 was 39,090 MW: 9,057 MW identified and 30,033 MW undiscovered (USGS, 2008). Currently, the potential hydrothermal capacity is 31,376 MW, comprising 5,566 MW of identified and 25,810 MW of undiscovered resources. The potential capacity for the identified resources is based on available reservoir thermal energy information from studies conducted at the sites (USGS, 2008); (Williams et al., 2009), subsequent research on plant additions and retirements conducted for the 2021 market report (Robins et al., 2021), and input from plant operators. Therefore, the identified resource capacity is the capacity available for future deployment and not the mean capacity estimated in the initial 2008 USGS assessment. The current undiscovered hydrothermal resource estimate is based on a series of geographic information system statistical models of the spatial correlation of geological factors that facilitate the formation of geothermal systems (Williams et al., 2009), excluding sites in federally protected lands.
For current total capacity estimations, the original USGS resource potential estimates for hydrothermal are updated with the following modifications:
- Installed capacity of about 3.7 gigawatts (GW) is excluded from the resource potential used in the 2019 GeoVision study (DOE, 2019); (Robins et al., 2021).
- 90 MW of net capacity have been brought online since 2016 and are not included in the 2024 ATB resource potential (Robins et al., 2021).
- Resources on federally protected and U.S. Department of Defense lands, where development is highly restricted, are excluded from the resource potential, as are resources on lands where significant barriers that prevent or inhibit the development of geothermal projects were identified by Augustine, Ho, and Blair (Augustine et al., 2019).
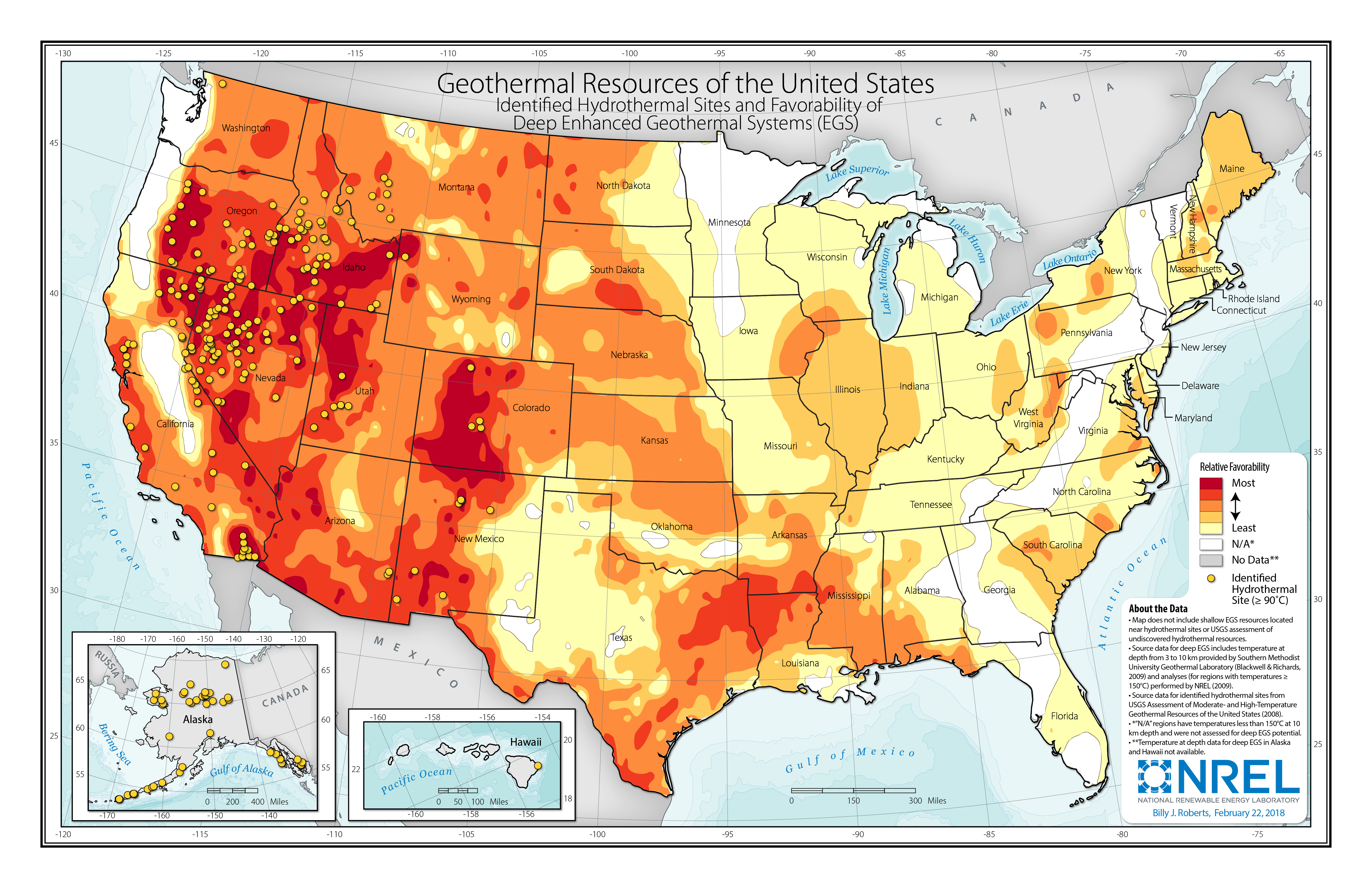
The EGS resource potential is concentrated in the western United States, but technology innovations as described in the Advanced Scenario and in the recent Enhanced Geothermal Shot initiative are anticipated to increase potential beyond the western United States (Augustine et al., 2023). The total recoverable potential is greater than 100,000 MW: 1,494 MW of NF-EGS and the remainder from deep EGS. The NF-EGS resource potential is based on data from the USGS for EGS potential on the periphery of select identified hydrothermal sites (Augustine et al., 2019). The deep-EGS resource potential ((Roberts, 2018); (Augustine, 2016)) is based on Southern Methodist University Geothermal Lab temperature-at-depth maps, and the methodology is from (MIT, 2006). The deep-EGS favorability map above and many other geothermal resource maps can be created using data from NREL's Renewable Energy Atlas (formerly the Geothermal Prospector tool).
Scenario Descriptions
Scenario | Drilling Advancements | EGS Development |
---|---|---|
Conservative Scenario | Technology Description: Drilling efficiency improvements (e.g., using mechanical specific energy with polycrystalline diamond compact bits and limiting bit dysfunction leads to longer bit life) result in minor decreases in drilling costs and little to no timeline reduction. Justification: Substantial increases in drilling ROP are unlikely without wider adoption of oil and gas technologies and new bit innovations. | Technology Description: Current well stimulation techniques do not consistently generate adequate economic flow rates of sustained flow from unsuccessful wells, which lead to little to no improvement of drilling success rate or CAPEX reduction. Justification: Stimulation is cost-prohibitive and lacks zonal isolation. Both the precision and scale of stimulation must improve. |
Moderate Scenario | Technology Description: ROP and bit life are doubled. Timelines and consumption of drilling materials are reduced. Justification: Cost modeling of drilling improvements along with limited successful field demonstrations and abundant oil and gas experience confirm this level of advancement is achievable (Lowry et al., 2017a); (Lowry et al., 2017b); (Hackett et al., 2020); (El-Sadi et al., 2024); (Dupriest and Noynaert, 2024). | Technology Description: As in the Conservative Scenario, although stimulation success has progressively improved, stimulation techniques remain cost-prohibitive. Justification: To remain consistent with the GeoVision report (DOE, 2019), cost modeling for stimulation technology has yet to be performed for a Mid Case scenario. In addition, successful deployment of EGS technology is modeled as coupled with significant drilling advancements because lower drilling costs and improved directional drilling in hard rock environments will likely help enable EGS reservoir development. |
Advanced Scenario | Technology Description: ROP and bit life are increased fourfold over the Conservative Scenario. Wells are constructed as monobore wells using expandable casing. The increased speeds result in significantly shorter timelines and lower consumption of drilling-related materials. Justification: Ongoing Advanced Research Projects Agency-Energy, Sandia National Laboratories, NREL, and other research (e.g., laser drilling, millimeter wave, and electric pulse research) are directed at reducing the cost and duration of well drilling. Growing interest from the oil and gas sector is leading to knowledge transfer. Monobore wells are already being drilled in that sector. | Technology Description: Stimulation success rate, control, and sustained flow rate advance to economic levels. EGS power plants are built with 100 MW of capacity. Permitting timelines are reduced to reflect anticipated permit streamlining effects of a National Renewable Energy Coordination Office, as created in the Energy Act of 2020. Justification: EGS Collab, FORGE initiative, and other U.S. Department of Energy (DOE) Geothermal Technologies Office-sponsored research are demonstrating stimulation techniques in hard rock environments, including hydraulic shearing, zonal isolation, and other techniques. Also, EGS developments are not resource-constrained, so larger plants will be more economical to build and operate. |
Impacts |
|
|
References |
|
Scenario Assumptions
For the 2024 ATB, several assumptions are updated for the Conservative, Moderate, and Advanced Scenarios based on consultations with geothermal industry experts and stakeholders and an updated literature search (Snyder et al., 2017); (Fukui et al., 2017); (Latimer and Meier, 2017); (Kneafsey et al., 2022); (Norbeck et al., 2023); (Norbeck and Latimer, 2023); (El-Sadi et al., 2024). Overall, the plant contingency factor for all technologies remains at the 10% level set in the 2023 ATB to ensure consistency with other thermal energy systems (Theis, 2021). This plant-level factor does not include the contingency for the drilling and completions that is already accounted for in the Well Cost Simplified model (Lowry et al., 2017b). The drilling costs for the Conservative Scenario for all technologies decrease from previous levels by 7% to match industry learnings as described in (El-Sadi et al., 2024). This is in addition to the 3% drilling cost reduction from the GeoVision baseline that had been applied in the 2021 ATB, resulting in a total 10% decrease from the GeoVision baseline well cost curve. The Moderate and Advanced hydrothermal assumptions are not changed from their 2023 ATB values.
Several EGS assumptions have also been updated in the Geothermal Electricity Technology Evaluation Model (GETEM), including the following:
- Successful full-size exploration wells: Remain at 2023 ATB values of five wells for Conservative, three for Moderate, and two for Advanced EGS scenarios.
- Exploration drilling success rate: Up from 76% to 80% and from 52% to 60% for the Moderate NF-EGS and Deep EGS scenarios, respectively. Up from 90% to 95% for both the Advanced NF-EGS and Deep EGS scenarios. This is based on horizontal well drilling successes in EGS projects in Nevada and Utah (Norbeck et al., 2023); (El-Sadi et al., 2024) and consultations with industry stakeholders.
- Development drilling success rate: Up from 76% to 80% for both NF-EGS and Deep EGS Moderate scenarios. Up from 90% to 95% for both NF-EGS and Deep EGS Advanced scenarios. This is based on horizontal well drilling successes in EGS projects in Nevada and Utah (Norbeck et al., 2023); (El-Sadi et al., 2024).
- Stimulation success rate: Updated from 75% (2023 ATB) to 80% (2024 ATB), 81% to 85%, and 90% to 95% for the NF-EGS and Deep EGS Conservative, Moderate, and Advanced scenarios, respectively, in accordance with industry trends (Norbeck and Latimer, 2023); (Pengju Xing et al., 2024).
- Flow rate per production well: Updated from 40 kilograms/second (kg/s) in the 2023 ATB to 60 kg/s for NF-EGS and Deep EGS Conservative scenarios to match recent industry trends (Norbeck et al., 2023); (Norbeck and Latimer, 2023).
- Productivity/injectivity index: Updated productivity and injectivity indexes from 2023 ATB values to match reservoir performance levels required for commercial operations (Norbeck and Latimer, 2023). See table below.
- Plant size: Aligned the baseline plant sizes of Deep EGS (flash and binary) with the NF-EGS values. Increased the 2023 ATB EGS Binary Moderate plant sizes from 30 megawatts-electric (MWe) (NF-EGS) and 25 MWe (Deep EGS) to 40 MWe each to account for trends in commercial field development plans.
GETEM Input Parameter | NF-EGS | Deep EGS | |||||
Conservative | Moderate | Advanced | Conservative | Moderate | Advanced | ||
Exploration | Successful full-size wells | 5 | 3 | 2 | 5 | 3 | 2 |
Drilling and Completion | Drilling cost curve | Baseline x 90%* | Intermediate I | Ideal | Baseline x 90%* | Intermediate I | Ideal |
Success rate (exploration) | 76% | 80% | 95% | 52% | 60% | 95% | |
Success rate (development) | 76% | 80% | 95% | 76% | 80% | 95% | |
Success rate (stimulation) | 80% | 85% | 95% | 80% | 85% | 95% | |
Reservoir Performance | Flow rate per production well | 60 kg/s | 80 kg/s (binary) 60 kg/s (flash) | 110 kg/s (binary) 80 kg/s (flash) | 60 kg/s | 80 kg/s (binary) 60 kg/s (flash) | 110 kg/s (Binary) 80 kg/s (Flash) |
Productivity index | 1,365 lb/hr-psi | 2,500 lb/hr-psi | 6,370 lb/hr-psi | 1,365 lb/hr-psi | 2,500 lb/hr-psi | 6,370 lb/hr-psi | |
Injectivity index | 1,650 lb/hr-psi | 3,000 lb/hr-psi | 7,645 lb/hr-psi | 1,650 lb/hr-psi | 3,000 lb/hr-psi | 7,645 lb/hr-psi | |
Plant Size | Power sales | 30 MWe (binary) 40 MWe (flash) | 40 MWe | 100 MWe | 30 MWe (binary) 40 MWe (flash) | 40 MWe | 100 MWe |
* 7% reduction based on most recent drilling cost data in addition to the 3% reduction from the GeoVision Baseline implemented in the 2021 ATB = 10% overall reduction.
Estimated bottom-up costs for the representative plant in the Moderate and Advanced Scenarios are expected to set in at the full deployment year (i.e., 2035). Between 2022 and 2035, learning rates are applied to account for annual learning-by-doing-based capacity deployments. In the 2023 ATB, separate learning rates for hydrothermal and EGS technologies were established through an NREL literature review. This literature review examined the application of one-factor, two-factor, and multifactor learning curves in geothermal, unconventional oil and gas drilling, and other renewable energy technologies. A one-factor learning curve, with a learning rate representing industrywide learning, is used in the ATB cost projections (Fukui et al., 2017). As in the 2023 ATB, a 13% learning rate is used to create the Moderate hydrothermal cost curve between 2022 and 2035 based on historical learning rates in the unconventional oil and gas industry (Fukui et al., 2017). EGS has a higher learning rate than hydrothermal systems because it is a novel technology and will likely be implemented in less predictable and more heterogeneous lithology. An 18% learning rate is used in the Moderate EGS cost curves, reflecting the low-end approximation for learning rates found in (Latimer and Meier, 2017). The Advanced hydrothermal cases use the high-end estimation of learning rates of 30% found in (Latimer and Meier, 2017). This learning rate also falls within the range predicted in a recent report by (Schulz and Livescu, 2023) on the effect of the full extent of oil and gas learning spillover into geothermal. The learning rate for Advanced EGS cases is increased from its 30% value in the 2023 ATB to 35% to reflect the learning seen in industry EGS projects (El-Sadi et al., 2024). After 2035, the costs in the Moderate and Advanced Scenarios are assumed to decrease annually by 0.5%, just as in the Conservative case.
Scenario | Base Year–2035 | 2035–2050 |
Conservative Scenario | 0.5% annual cost reduction | 0.5% annual cost reduction |
Moderate Scenario | 13% (hydrothermal), 18% (EGS) | 0.5% annual cost reduction |
Advanced Scenario | 30% (hydrothermal), 35% (EGS) | 0.5% annual cost reduction |
The learning curve equation used to determine future costs (between 2022 and 2035) is the single-factor learning equation as described by (Fukui et al., 2017): $$C(x) = C(x_{0})(x/x_{0})^{-L}$$ where C(x) is the cost at cumulative capacity x, C(x0) is the cost at the first deployment capacity x0, and L is the learning parameter. The learning rate (LR) and L are defined by the following relationship: \(LR=1-2^{-L}\). To convert from capacity to time, the relative capacity, x/x0 at the full deployment year (2035), is first determined using the known 2035 cost and the LR for the specific scenario. Then a simple linear interpolation is applied between 2022 (when x/x0 = 1) and 2035 to determine the x/x0 at intermediate time steps. These values are then applied in the learning curve equation to calculate C(x) for the corresponding years.
Scenario | ROP (ft/hr) | Bit Life (hr) | EGS Flow Rate (kg/s) |
---|---|---|---|
Conservative Scenario (2022)* | 25 | 50 | 60 |
Moderate Scenario | 50 | 100 | 60 flash/80 binary |
Advanced Scenario | 100 | 200 | 80 flash/110 binary |
* The 10% (3% in 2021 ATB plus 7% in 2024 ATB) reduction in the baseline drilling cost would correspond to a slightly higher ROP and longer bit life than the GeoVision baseline assumptions displayed here. Updated analysis to create a new baseline cost curve in the Well Cost Simplified model will be implemented in a future ATB cycle using updated industry drilling performance values (Norbeck and Latimer, 2023).
Representative Technology
Hydrothermal
Hydrothermal geothermal technologies encompass technologies for exploring for the resource, drilling to access the resource, and building power plants to convert geothermal energy to electricity. Technology costs depend heavily on the hydrothermal resource temperature and well productivity and depth to the point that project costs are site-specific and a "typical" cost applied to any given site would be inaccurate. The 2024 ATB uses scenarios developed by the DOE Geothermal Technologies Office (Mines, 2013) for representative binary and flash hydrothermal power plant technologies.
The first scenario assumes a 175°C resource at a depth of 1.5 km with wells producing an average of 110 kg/s of geothermal brine supplied to a 30-MWe binary (organic Rankine cycle) power plant. The second scenario assumes a 225°C resource at a depth of 2.5 km with wells producing 80 kg/s of geothermal brine supplied to a 40-MWe dual-flash plant. These are midgrade or "typical" temperatures and depths for binary and flash hydrothermal projects.
The 2024 ATB representative technologies fall in the middle to low end of the hydrothermal resources cost estimates typically deployed in Regional Energy Deployment System (ReEDS) model runs.
Enhanced Geothermal Systems
As with costs for projects that use hydrothermal resources, EGS resources are similarly site-specific and project costs depend heavily on the resource temperature, well productivity, and depth. The 2024 ATB uses scenarios developed by the DOE Geothermal Technologies Office (Mines, 2013) for representative binary and flash Deep-EGS power plants assuming current EGS technology performance metrics (Norbeck and Latimer, 2023). NF-EGS representative scenarios mimic the temperature, depth, and plant size of the hydrothermal representative plants, but they include EGS techno-economic assumptions such as flow rate, success rate, and well productivity/injectivity. In the context of the GETEM model, NF-EGS systems are assumed to be brownfield projects with lower exploration cost requirements than greenfield Deep-EGS developments.
The first NF-EGS scenario assumes a 175°C resource at a depth of 1.5 km with wells producing an average of 60 kg/s of geothermal brine supplied to a 30-MWe binary (organic Rankine cycle) power plant. The second NF-EGS scenario assumes a 225°C resource at a depth of 2.5 km with wells producing 60 kg/s of geothermal brine supplied to a 40-MWe dual-flash plant. These NF-EGS representative scenarios fall close to the average resource cost estimates seen in the NF-EGS supply curves.
The first Deep-EGS scenario assumes a 175°C resource at a depth of 3 km with wells producing an average of 60 kg/s of geothermal brine supplied to a 30-MWe binary (organic Rankine cycle) power plant. The second Deep-EGS scenario assumes a 250°C resource at a depth of 3.5 km with wells producing 60 kg/s of geothermal brine supplied to a 40-MWe dual-flash plant. These temperatures and depths are at the low-cost end of the EGS supply curve and would be some of the first developed.
Methodology
This section describes the methodology to develop assumptions for CAPEX, O&M, and capacity factor. For standardized assumptions, see labor cost, regional cost variation, materials cost index, scale of industry, policies and regulations, and inflation.
The site-specific nature of geothermal plant cost, the relative maturity of hydrothermal plant technology, and the very early-stage development of EGS technologies make cost projections difficult. The GeoVision scenarios are based on bottom-up analysis of potential cost and performance improvements. The inputs for these scenarios were developed by the national laboratories as part of the GeoVision effort and were reviewed by industry experts.
The cost and performance estimates are calculated using the Geothermal Electricity Technology Evaluation Model (GETEM), a bottom-up cost analysis tool that accounts for each phase of development of a geothermal plant as follows:
- Cost and performance data for hydrothermal generation plants are estimated for each potential site using GETEM. Model results are based on resource attributes (e.g., estimated reservoir temperature, depth, and potential) of each site.
- Site attribute values are from (USGS, 2008) for identified resource potential and from capacity-weighted averages of site attribute values of nearby identified resources for undiscovered resource potential.
- GETEM is used to estimate CAPEX, O&M, and parasitic plant losses that affect net energy production for the three technology innovation scenarios.
Capital Expenditures (CAPEX)
Definition: For the ATB—and based on (EIA, 2016) and GETEM component cost calculations—the geothermal plant envelope is defined to include the following:
- Geothermal generation plant
- Exploration, confirmation drilling, well-field development, reservoir stimulation (EGS), plant equipment, and plant construction
- Power plant equipment, well-field equipment, and components for wells (including dry/noncommercial wells).
- Balance of system
- Installation and electrical infrastructure, such as transformers, switchgear, and electrical system connecting turbines to one another and to the control center
- Project indirect costs, including costs related to engineering, distributable labor and materials, construction management startup and commissioning, and contractor overhead costs, fees, and profit.
- Financial costs
- Owners' costs, such as development costs, preliminary feasibility and engineering studies, environmental studies and permitting, legal fees, insurance costs, and property taxes during construction
- As in the 2023 ATB, the 10% contingency factor is included in the overall capital cost calculations (Theis, 2021); this contingency factor is not applied to drilling costs because drilling success rates and contingencies are already incorporated into the GETEM model
- Electrical interconnection and on-site electrical equipment (e.g., switchyard), a nominal-distance spur line (<1 mile), and necessary upgrades at a transmission substation; distance-based spur line costs (grid connection costs, or GCC) are now included the ATB
- Interest during construction estimated based on 5-year durations for hydrothermal and EGS for the Advanced Scenario, 7-year durations for hydrothermal and EGS for the Moderate Scenario, and 8-year and 10-year durations for hydrothermal and EGS, respectively, for the Conservative Scenario, accumulated at different intervals for hydrothermal and EGS based on schedules as outlined by the GeoVision analysis (found at www.energy.gov/eere/geothermal/geovision) (ConFinFactor).
In the 2024 ATB, CAPEX is shown for six representative plants. If all sites are used, the CAPEX estimates for all hydrothermal and NF-EGS potential result in a CAPEX range much broader than that shown in the ATB. It is unlikely that the resource potential from all identified sites will be developed because of the high costs for some sites. Effects of regional cost variation and distance-based spur line costs are not estimated.
CAPEX in the ATB does not represent regional cost variants (CapRegMult) associated with labor rates, material costs, or other factors (CapRegMult = 1).
CAPEX in the ATB now includes an ATB-wide technology-agnostic spur line and interconnection (GCC) cost from plant to transmission grid (GCC = $100/kW). See the ATB Definitions page for more details.
Base Year: GETEM inputs are mostly derived from the business-as-usual scenario from the GeoVision report ((DOE, 2019); (Augustine et al., 2019)). Costs are for new or greenfield hydrothermal projects—not for redrilling or additional development/capacity additions at an existing site. The exception is for NF-EGS, where the brownfield hydrothermal assumptions are used in GETEM. The following chart shows historical CAPEX and levelized cost of energy (LCOE) for geothermal, including data from the International Renewable Energy Agency (IRENA, 2023).
Future Years: Projections of future geothermal plant CAPEX for three scenarios are derived from modeled costs in the GeoVision report (DOE, 2019) and based on updated learning rates following (Fukui et al., 2017); (Latimer and Meier, 2017):
- Conservative Scenario: Continuation of current industry trends in drilling (e.g., minor efficiency improvements with little to no increase in ROP) and EGS (e.g., subeconomic flow rates and limited stimulation reproducibility) result in a minimum learning rate and minor CAPEX improvements as implemented in the U.S. Energy Information Administration's Annual Energy Outlook 2015 (EIA, 2015): 10% CAPEX reduction by 2035 (from 2015). This corresponds to a 0.5% annual improvement in CAPEX, which is assumed to continue through 2050.
- Moderate Scenario: Drilling advancements, specifically the Intermediate 1 Drilling Curve (e.g., doubled ROP and bit life and reduced number of casing intervals, associated drilling materials, and timelines) detailed as part of the GeoVision report, result in cost improvements achieved by 2035. Stimulation success rates for EGS increase to 85% because of advances in zonal isolation during stimulation and improved fractured conductivity poststimulation (Kneafsey et al., 2022); (Norbeck et al., 2023). Well productivity indexes are increased to match values in (Snyder et al., 2017) and (Norbeck and Latimer, 2023). Costs decrease according to the learning curves described above from present values to the 2035 projected values followed by a 0.5% annual reduction in CAPEX, because of a minimum learning rate, through 2050.
- Advanced Scenario: Significant drilling and EGS advancements based on modeling in the Technology Improvement scenario of the GeoVision report result in cost improvements achieved by 2035. Costs decrease according to the learning curves described above from present values to the 2035 projected values and are followed by a 0.5% annual reduction in CAPEX, because of a minimum learning rate, through 2050. Drilling improvements, as part of the GeoVision Ideal Drilling Curve, include significantly increased ROP, bit life, and EGS stimulation success, limited casing intervals, and significantly reduced consumption of drilling materials. In addition, the deployment of 100-MW power plants decreases capital costs and fixed O&M costs. Well productivity indexes reflect high-end values found in (Snyder et al., 2017). Progressive EGS demonstrations and commercial projects result in significant advances in EGS exploration drilling success rate and minimal exploration wells required for resource confirmation: 95% and two successful exploration wells, respectively. Finally, permitting timelines are reduced to reflect anticipated permit streamlining effects of the National Renewable Energy Coordination Office, as enacted through the Energy Act of 2020.
Use the following table to view the components of CAPEX.
Operation and Maintenance (O&M) Costs
Definition: Fixed O&M (FOM) costs represent average annual fixed expenditures (and depend on rated capacity) required to operate and maintain a hydrothermal plant over its lifetime of 30 years (plant and reservoir), including the following:
- Insurance, taxes, land lease payments, and other fixed costs
- Present value and annualized large component overhaul or replacement costs over technical life (e.g., downhole pumps)
- Scheduled and unscheduled maintenance of geothermal plant components and well-field components over the technical lifetime of the plant and reservoir.
Base Year: GETEM is used to estimate FOM for each of the six representative plants. FOM costs for NF-EGS and EGS are equivalent. For the 2022 ATB, Base Year FOM costs were decreased by 23%. This change is carried over to the 2024 ATB and is supported by proprietary geothermal industry FOM data.
Future Years: Future FOM cost reductions are based on results from the GeoVision Technology Improvement scenario (DOE, 2019) and are described in detail by Augustine, Ho, and Blair (Augustine et al., 2019).
Use the following table to view the components of operating expenditures.
Capacity Factor
Definition: Geothermal plant capacity factor is influenced by diurnal and seasonal air temperature variation (for air-cooled plants), technology (e.g., binary or flash), downtime, and internal plant energy losses.
Estimates of capacity factor for geothermal plants in the ATB represent typical operation.
Base Year: The capacity factor estimates are developed using GETEM at typical design air temperature and are based on design plant capacity net losses. An additional reduction is applied to approximate potential variability because of seasonal temperature effects.
Some geothermal plants have experienced year-on-year reductions in energy production, but this is not consistent across all plants. No approximation of long-term degradation of energy output is assumed.
Future Years: Capacity factors remain unchanged from the Base Year through 2050. Technology improvements focus on CAPEX costs. The dispatch characteristics of these systems can be valuable to the electric system to manage changes in net electricity demand. Actual capacity factors will be influenced by the degree to which system operators call on geothermal plants to manage grid services. However, a constant dispatch profile is modeled in the ATB, and no change over time is assumed.
References
The following references are specific to this page; for all references in this ATB, see References.